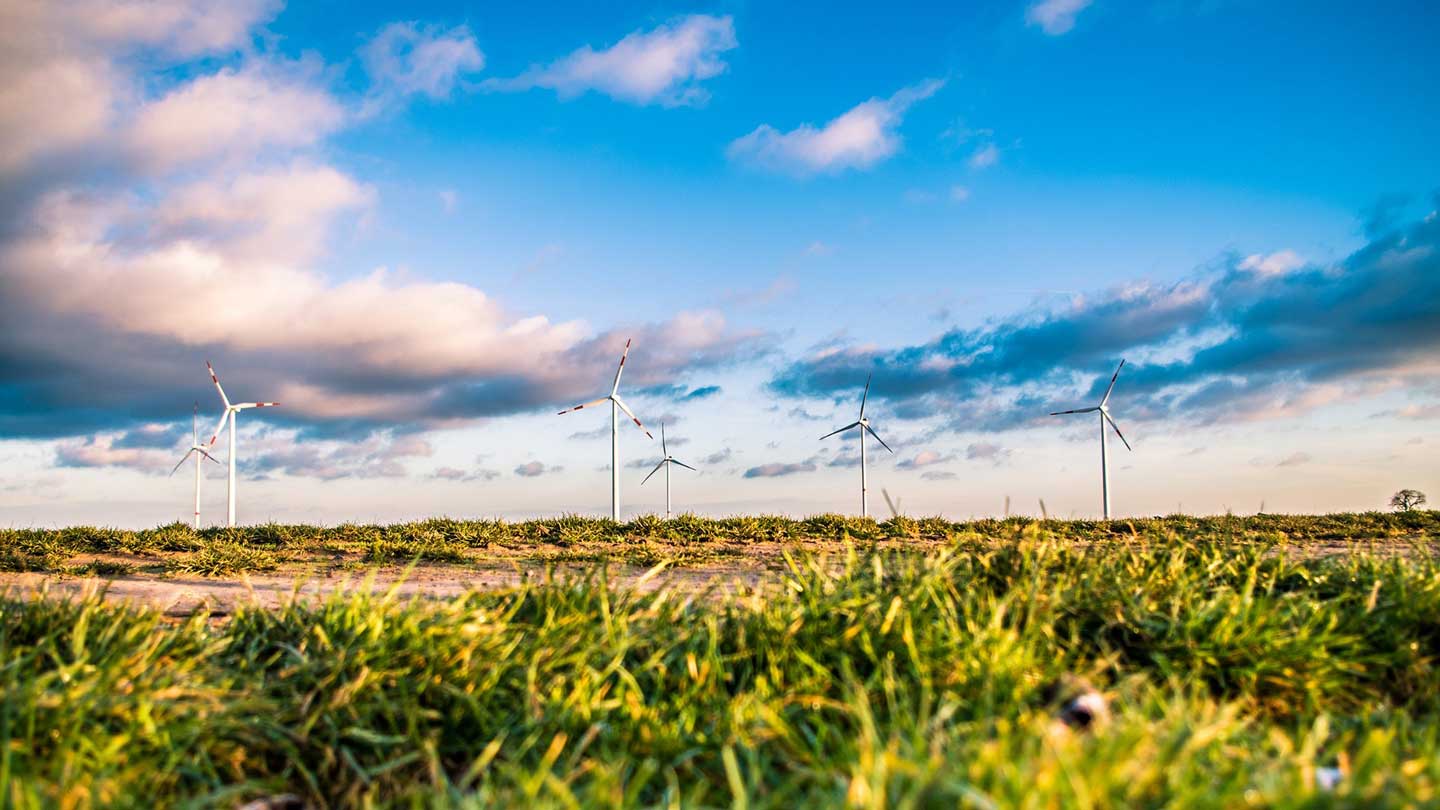
ALTERANTIVE ENERGY
INTRODUCTION
Alternative energy sources have been around for many years, although in the past the abundance of cheap fossil fuels and the relatively high cost of producing energy from alternative or ‘non-conventional’ sources meant that there has been limited commercial or government motivation to research or invest in alternative sources of energy.
Today, that situation has changed radically due to technology advances, greater global awareness of Climate change and a changed political environment. We believe that there are five principal drivers of the ongoing debate surrounding alternative energy. These are:
We believe that there are five principal drivers of the ongoing debate surrounding alternative energy.
These are:
- National energy security and the rise of ‘energy nationalism’
- High fossil fuel prices and the debate over the relative depletion of existing resources of Hydrocarbons and fossil fuels.
- The rapidly growing need globally for further energy.
- Growing concerns about global warming and a consequent drive to ensure reduction of greenhouse gas (GHG) emissions.
- The relative cost (both capital and operating expenditure) of alternative energy sources.
Energy security has until recently not been a key focus issue for most nations. However, the increasing depletion of indigenous fossil fuels (eg in the UK and the US) plus several largely unforeseen geopolitical and meteorological events over the past two to three years have focused the governments of the developed world on the uneven distribution of fossil fuels around the world, in particular oil and gas. This is especially true if one considers the balance of geographical energy requirements and fossil fuel distribution, with increasing transportation fuel and energy needs, especially in India, China, the US and the EU versus the availability of significant resources of oil and gas in Russia, the Caspian Sea region, Africa and the Middle East. Europe, for example, is becoming increasingly dependent on imported hydrocarbons.
The EU expects that in a ‘business as usual’ scenario, the EU’s energy import dependence will jump from 50% of total energy consumption today to 65% in 2030. Reliance on imports is expected to increase from 57% to 84% for gas by 2030 and from 82% to 93% for oil (source: EU White paper on Energy Policy). We note that the US is in a similar position, as today it imports over half of its oil (60.4% in December 2006 – source: EIA). Gas imports are minimal currently, although we expect that these will rise in time, as US demand for natural gas rises and reserves are depleted. By 2030, the US is expected to be an importer of 16% of its gas in the form of LNG.
MAJOR SOURCES AND OPPORTUNITIES
Nuclear
With oil prices relatively high and concern about the potential impact of fossil fuels on the environment, talk of a nuclear energy solution is enjoying a revival. This talk comes at a time when new technologies are available to make nuclear power safer and help deal with the issue of disposal of radioactive waste.
Advanatage
TNuclear energy has a number of positives going for it. First, it does not give off carbon emissions, earning it supporters in the environmental community among those concerned about global warming. Second, unlike oil, two of the three largest producers are Australia and Canada, both of which have stable governments and represent reliable sources of supply. Third, once reactors are built, it is very cost effective to keep them running at high capacity and for utilities to address demand fluctuations by cutting back on usage of fossil fuels. Fourth, nuclear plants tend to last a long time and many existing plants have become more efficient over time, reducing their demand for uranium.
Disadvantage
There are a number of disadvantages to the nuclear-power option. These include not only the obvious safety questions but also some economic and supply-related questions that are currently being debated by those for and opposed to renewal of outdated power plants or an expansion of the sector. In terms of safety, two issues are regularly debated. First, the issue of nuclear waste and, second, concerns over potential terrorist attacks on nuclear power plants. The first objection may be overcome through the introduction of new types of power plants, such as the pebble-bed modular reactor. This type of reactor uses graphite balls flecked with tiny amounts of uranium, rather than conventional fuel rods. With the fuel encased in graphite and impermeable silicon carbide, the theory is that the waste should be relatively easy to dispose of. The terrorism fears are less easily addressed and may ultimately stall the construction of new plants in countries such as the U.S., where these worries are greatest. Among economic concerns is the question of construction costs. Although the cost of energy produced by existing nuclear plants is competitive, the upfront capital costs of constructing new plants are extremely high, calculated at $1,300-$1,500 per kilowatt- hour, or twice the amount it costs to construct a gas-fired power station.10 In addition, nuclear plant operators are subject to a government tax to help pay for the disposal of nuclear waste, pushing potential costs even higher. Given the long life of nuclear power stations, however, supporters argue that the upfront costs, at least, are justified.
Another concern is the availability of the main fuel source, uranium. Having been stable for a number of years, uranium prices nearly tripled between March 2003 and May 2005. As in the case of oil, the source of this jump in prices can, in part, be traced to China. Both the Asia Pacific Foundation of Canada and the Uranium Information Center (UIC), an Australian-based organization, believe that more supply will have to be found to meet growing demand in Asia and in a revival of interest in the western world. Only 55 percent11 of supply currently comes from primary mine production, with the rest coming from military and other sources, such as reprocessed fuel from the power stations themselves. Both organizations believe that this percentage is likely to rise in the future, along with production.
Outlook
In many countries, concerns about safety, short-term economics and supply of uranium are likely to be outweighed by the desire to acquire a relatively non-polluting and secure source of power. China, for example, plans to build 30 new plants by 2050, generating as much as 300 gigawatts10 of power. In certain European countries, such as France and Finland, nuclear energy is a lot more popular than it is in the U.S.
DISTRIBUTED ENERGIES:INCREASING THE ATTRACTIVENESS OF ALTERNATIVES
The massive blackout across the northeastern United States in August 2003 did much to focus attention on the problems of the electrical grid structure in the U.S. It served to increase interest in different ways of looking at electricity transmission, including providing the grid with storage capacity and distributed energy; that is, localized energy production and distribution. Such localized power production may ultimately encourage swifter adoption of alternative energy sources.
Energy Storage
Storage of electricity through conversion to another fuel is expensive. Nevertheless, higher initial costs are typically offset over the longer term due to the efficiencies storage promotes. Storage also provides flexibility through the peaks and troughs in demand, as well as supply. To deal with fluctuations in demand, energy storage is most commonly used in conjunction with nuclear power. Nuclear plants need to operate flat out, which often means that excess electricity is generated overnight. If a nuclear plant is paired with a hydroelectric plant, and the surplus energy generated during trough demand is used to pump water back up behind the hydroelectric dam, this energy can be stored and released as hydroelectric power during peak demand periods.
One of the criticisms often leveled at alternative energy sources such as wind and solar power is that they are intermittent in nature. Using wind or solar energy to generate hydrogen converts these types of energy sources into other fuels, helping to bridge the gap when, for instance, the wind is not blowing or the sun is not shining.
Symbiotic pairs include:
Coal plus oil field for carbon storage.
Nuclear plus hydroelectric.
Wind/solar plus hydrogen production.
Hydro Energy : Biggest Alternative Source
Hydro is the biggest alternative energy source currently. The IEA estimates that hydro is currently the biggest alternative energy electrical power generation source, accounting for around 20% of global electricity generated. However, the market for hydro power is relatively mature. The IEA estimates that globally around 60% of available hydropower resources have already been exploited./p>
Biomass and biofuels
Biomass refers to a number of different processes whereby living matter or its metabolic byproducts (eg manure) are converted into energy. Most commonly, biomass is converted into biofuel, but biomass can include biodegradable waste, which can be burnt for heat/power or turned into methane. This has vast potential in many countries. Biofuels include bioethanol, biodiesel, biobutanol and biogas.
Like coal and petroleum, biomass is a form of stored solar energy. Essentially, biomass is part of the carbon cycle. Carbon from the atmosphere in the form of CO2 is converted into biological matter through photosynthesis. Biomass is converted into fuel in a biofuels plant, which is then in turn combusted in an engine, releasing the carbon once more into the atmosphere, where it is once more used by plants to create biological matter through photosynthesis. This is important because in essence biofuels are not emission less. When combusted, most biofuels do not emit sulphur, nitrogen or other impurities and thus are ‘clean’ in the sense that they produce only CO2.
Sourcing fuels from biological sources is critical to the perception and regulatory support of biofuels. This is because the biomass from which they are produced removes CO2 from the atmosphere and the use of biofuels reduces carbon and other emissions by displacing the combustion of traditional fossil fuels. Different types of biomass absorb different amounts of CO2 when growing and therefore the type of biomass used will impact the amount of carbon offset for each type of plant. We believe that only the conversion of Brazilian sugarcane to bioethanol actually results in a net reduction in overall CO2, including the energy expended to convert the sugar cane to fuel. For most other types of biomass and most other biofuels, we believe that the carbon balance is less favourable, either because of the amount of carbon consumed by the biomass is low or more importantly the energy needed to convert the biomass into biofuel is higher than for sugar cane. Typically, types of biomass include corn and soybean in the US and primarily rapeseed and flaxseed in Europe. Sugarcane is the main biomass in Brazil and palm oil in Southeast Asia. These types of biomass are used to produce principally biodiesel and bioethanol. Agricultural and household waste such as straw, wood chippings, sewage and food can be used to make biogas. The production of biofuels clearly has a seat at the energy table as a means of ensuring security of national transportation fuel, especially for those countries such as the US, Brazil, Europe, which are increasingly reliant on imported oil and gas. The manufacture of biofuels is clearly more expensive (by a factor of around 2.5 times) than wind in USD/kW of output. However, emissions reduction and energy security are driving favourable legislation, subsidies and tax breaks to encourage the industry, especially in Europe but also in the US. We believe that biofuels have a future, although we note that a number of serious issues face the industry, including:
Solar Energy
Solar power involves converting the sun’s energy into heat or electricity via solar photovoltaic (PV), concentrated solar power or solar thermal systems. Solar power is currently experiencing prolific growth and should continue to form a small but growing part of the energy matrix – not only solar power for distributed power sources (domestic etc) but also to some extent for commercial solar “farms”. As for so many of the renewable or alternative energy sources, the key mantras remain energy security and emission reductions.
Solar – initial usage for domestic and small business?
We believe that solar will find its biggest applications in domestic and small business usage, as the high cost of solar will discourage most utility-scale operations, while we expect that the land usage of solar facilities will militate against most commercial facilities, apart from on the tops of buildings. While solar power cannot be generated at cost parity with wholesale electricity prices, its cost is comparable to the retail price of electricity. Given the cost and the fact that electricity generated is DC, this gives it potentially lots of domestic applications. Solar Century, a UK-based installer of domestic solar tiles on roofs, estimates that eight m2 are required to provide 1kW of electrical output, which means that most average three bedroom houses could support around 2kW of production capability and that you get about 125W from each m2 of PV cell.
Solar too expensive for utility-scale facilities?
Most commercial facilities are simply too small for widespread utility scale power generation and also they would take up too much space. The world’s largest solar farm is in Bavaria with a capacity of just 10MW. It occupies 63 acres, suggesting that it generates just 40W of power per m2. The first commercial-scale plant is being planned in New Mexico – a 300MW plant. However, the cost is estimated to be USD1.6bn, or USD5,333 per kW, versus around USD1,400 per kW for wind and around USD500 per kW for gas-fired generation capability.
(We analyze in more detail the relative cost position of the different technologies in the chapter entitled ‘Cost comparison of different sources of power generation’.)In addition, the plant will occupy a staggering 3,200 acres, or 12.25km2. By comparison, assuming mains gas, we estimate that the average 750MW rated CCGT would occupy about 350,000m2. This would therefore mean that you could fit 38 CCGT plants with a generation capability of around 28GW, or nearly 100 times more power, if a gas turbine were installed rather than solar PV, at a cost of USD14bn or just 10 times the cost of the solar farm. For a comparison with wind, we estimate that one 3MW wind turbine requires roughly 14 acres of land. Turbines in a park need to be sighted between 3-5 rotor diameters apart in the direction perpendicular to the prevailing wind and between 5-9 rotor diameters apart in the prevailing wind direction. This space is greater so as to optimize the turbine’s efficiency; as the turbulent air created by the turning of the rotor needs around 5-9 rotor lengths to return to being “clean” again Turbulent air would affect the efficiency of a turbine behind any turbine upwind of it. Thus, to make the same comparison for wind versus solar, on our 3,200 acre site you could locate 230 3MW turbines, generating just short of 700MW of electricity, i.e. more than twice as much as if the land were dedicated to solar, at a cost of USD700m or 44% of the solar farm. The beauty of course of wind power relative to conventional power generation is that 98% of the land can be returned for farming usage (if indeed the land is arable), whereas with solar or CCGT/coal, the land can have no other use apart from power generation. Aesthetes may argue about the industrialization of the arable landscape, although we think farmland with a few windmills on it is preferable to 12km2 of solar panels or 38 CCGT plants either floating or fixed, either to the ocean floor or to piles. This form of power generation has also been referred to as damless hydropower. Tidal power Tidal currents can also be harnessed by creating a dam or barrage across a river mouth, estuary or bay, such as the plant situated at La Rance in France. However, we do not foresee a substantial future for these types of project as the environmental impact is too great. In any case, if you can put a tidal turbine in a river mouth, why would you dam the whole river mouth? The pros of marine/tidal power All forms of marine and tidal power are still in the very early stages of development but we believe that converters of marine/tidal energy have huge prospects, based on the following notions: _ The relative predictability of tides means that the capacity load factor of marine turbines would necessarily be much higher than that of wind turbines _ Environmental issues such as noise and visual impact are not present for marine/tidal turbines as they are underwater.
Geothermal Energy
Geothermal energy is generated from heat that comes from the earth, and is used as a heat source or a power source. The best areas for utility-sized plants are where there is seismic and magma tic activity as well as young volcanism. In areas with high-temperature ground water at shallow depths wells are drilled into natural fractures in basement rock or into permeable sedimentary rocks. Hot water or steam flows up through the wells, either by pumping or through boiling (flashing) flow The steam produced is used to drive a turbine, thereby generating electricity. Medium enthalpy geothermal may have large scale heating applications rather than power. Around 25 countries make extensive use of geothermal energy, especially the US, the Philippines, Italy, Mexico and Iceland.
The relatively widespread nature of available resources, despite the attractive cost position of geothermal, does not make it a globally viable technology. However, as noted above, the availability of resources in certain specific locations makes it an interesting niche application, although not a power source that we expect to have global commercial application. We estimate that globally there is in the region of around 13GW of geothermal power capacity.
Wind Power
Wind-generated electricity accounted for 0.6% of global generation in 2004 and the IEA expects this to grow to 2.4% in 2014. The Global Wind Energy Council (GWEC) hopes that wind power will provide 12% of total power generation by 2020, although we believe that this is an aggressive target.
Key wind industry conclusions
Specifically on the wind industry our conclusions are that:
_ Wind is currently the most cost-competitive renewable energy technology. Pricing and shortages of raw materials and components have masked the reduction in terms of overall costs
_ Wind power is comparable to conventional fossil fuel sources from a cost position with oil prices over USD50. Given its emission profile, we therefore expect strong growth for wind
_ Wind remains a massively underutilized resource, with only 7% of available onshore resources utilized in Europe alone, within the current the grid infrastructure
_ This ignores the vast potential for offshore as well as further onshore potential in the event of grid upgrades
_ We forecast industry growth in the global market for wind turbine generators (WTG) of a five-year CAGR of 14% over 2006-10, despite some growth constraints on key component supplies
_ Strong demand for wind turbines is being driven by the entrance of utility players (rather than eco-individuals and private companies) into the renewable energy market.
Conclusion.
The results show that onshore wind and geothermal are competitive at current fossil fuel prices. Both are cheaper than coal and equivalent to gas on a fully costed EUR/MWh basis – only CCGT and nuclear are cheaper. We have observed the following:
The capital cost of geothermal and wind are among the highest but the only cost going forward is operating & maintenance cost as the fuel is essentially free
Coal- and gas-based technologies look very cheap on a capital cost only basis but in fact the bulk of the ultimate cost of the power generation is in the fuel and to a lesser extent the carbon costs. Natural gas-based turbine technology is less sensitive to carbon prices as the emission of CO2 is around half that of coal-fired plant.
Offshore wind still has a long way to go to become cost-competitive. Solar and fuel cells have even further to go. Wind, geothermal and solar have relatively predictable compared with any fossil fuel driven sources of power generation, as the raw material costs are zero, whereas all fuel based generation systems have uncertainty and volatility of oil, gas and coal prices Wind and Solar need to be used in conjunction with base load plants, as meteorological conditions are intermittent. We are therefore seeing the development of hybrid wind/gas and other such plants to cope.